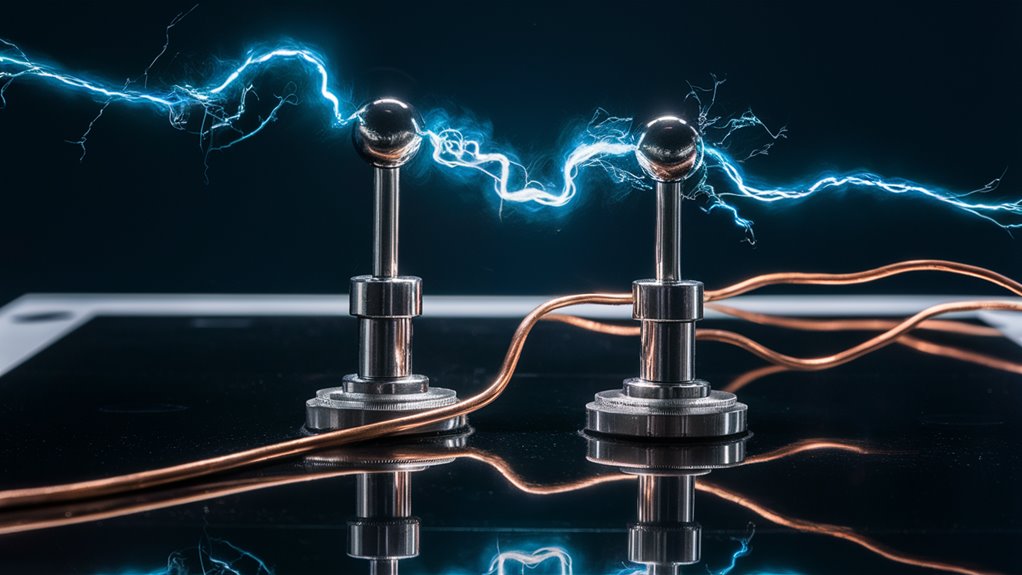
Ion Whip Systems: Advanced Particle Control in Electro-Magnetic Fields
The revolutionary potential of ion-lash technology resides in thoroughly controlled electromagnetic fields manipulating charged particle streams in ways entirely unimaginable before these advanced techniques were developed. The heart of this pioneering system relies on voltage differentials and the binding energies that occur only when precisely controlled fields are used for ever more exact benchwork of ions.
Quantum Control & Applications
Ion-lash systems have an inherent simplicity: they utilize electromagnetic forces at the quantum level. This makes it usable for nanofabrication as well as in medicine. Taking ionic manipulation quite seriously, the fine-table-binding force interactions of these systems are used for extremely precise particle manipulation, serving as an introduction to molecular engineering and quantum computing technologies.
New Trends in Technological Research and Development
The impact of free change in charge manipulation goes far beyond today. As new capabilities continue to emerge from the basic systems that constitute ion-lash technologies, multiple scientific fields stand to benefit immeasurably. Tying together quick table binding techniques with advanced particle control systems presents a potential quantum leap in both quantum and molecular manipulation.
Breaking New Ground in Particle Physics
The study of ion-lash dynamics takes a big step toward understanding behavior in charged particle systems. The ability of this technology to achieve precision control through electromagnetic field manipulation places it with the world’s best for new developments, although only application can deduce theoretical fruits so far. Where will these yield, then? Of course, some applied areas, primarily medical treatment and physics theory.
The Physics of Ion-Lash Theories
Fundamentals of Ionic Manipulation
Ionic manipulation serves as the basis of ion-lash theory, a process undertaken through exact application of coherent magnetic fields to charged particles. These fields produce regulated ionic streams which act like microscopic whips responding constantly to voltage differentials; force application patterns are consistently generated in advance.
Ion-Lash Mechanics
Charged particle streams in ion-lash systems are directed through special electromagnetic channels thanks to a technique’s development which can be compared with fine electric separating fibers.
Particles accelerate based on their charge-to-mass ratio, and their paths are set by the electromagnetic fields that are applied to them.
Field strength modulation and voltage adjustment allow for precise control of strengths and motion directions in a stream.
Material Interaction Characteristics
Interactions between ions and materials produce particular effects through the collective action of kinetic energy and electrical charge. This process creates certain models for broken molecular orientation, leading to reliable prediction and handling of results.
The great breakthrough is the concept of the matterflow binding sites, particle swarm of hitherto unknown force networks which are in harmony from the very beginning and can serve to bind, separate or change matter at a molecular level.
Major Tech Equipment:
Manipulation of electromagnetic fields
Acceleration of charged particles
Control of voltage differentials
Application of molecular-level forces
Synchronization of multiple streams
Quantum States During Particle Linking
Understanding Quantum States Within Slantwise Gains Particle Linking Processes
Quantum states during binding sequences between particles take on characteristic, predictably distinct forms as a result of ion-lash interactions.
Particles entering binding phases exhibit superposition state oscillations at frequencies directly proportional to their valence setups.
These quantum fluctuations produce bonding windows, critical periods in which particle coupling can take place.
Three Essential Stages of Quantum Bonding
Stage 1: Approach State
Particles maintain discrete quantum numbers while their wave functions start to overlap. This initial interaction phase sets up the groundwork for possible binding outcomes.
Stage 2: Critical Binding States
Quantum tunneling allows electrons to change between energy levels, making this a crucial stage in the binding process. During this stage, particles are at their greatest capacity for forming molecular bonds.
Stage 3: Coupled States
Shared electron clouds give birth to new quantum configurations, so that stable molecular structures arise from redistribution of electron clouds.
Predictive Patterns in Quantum Bindings
Vousvoyance collapse patterns serve as a reliable indicator for predicting the outcome of a link. By way of systematic study into ion-lash theory, these patterns show what lies behind selective linking or repulsion between particles.
They are also directly related to the strength and lifespan of the subsequent chemical bonds, making them capable of regulating binding processes in very fine detail.
By virtue of this quantal nature of quantum states, molecular formation can be predicted accurately and made to take place exactly under lab conditions, leading to a better understanding on particles in fundamental particle interactions.
Measuring Microsecond Ionic Interactions
New Methods for Measuring Microsecond Ionic Interactions
High-Resolution Detection Systems
Hi-tech microsecond ionic measurement systems are based on ultrafast laser spectroscopy and advanced time-resolved detection.
These high-end systems demand that all detection channels work in sync with sub-microsecond precision.
Photomultiplier tubes specially coupled to high-bandwidth oscilloscopes achieve close tracking of quick charge-state transitions.
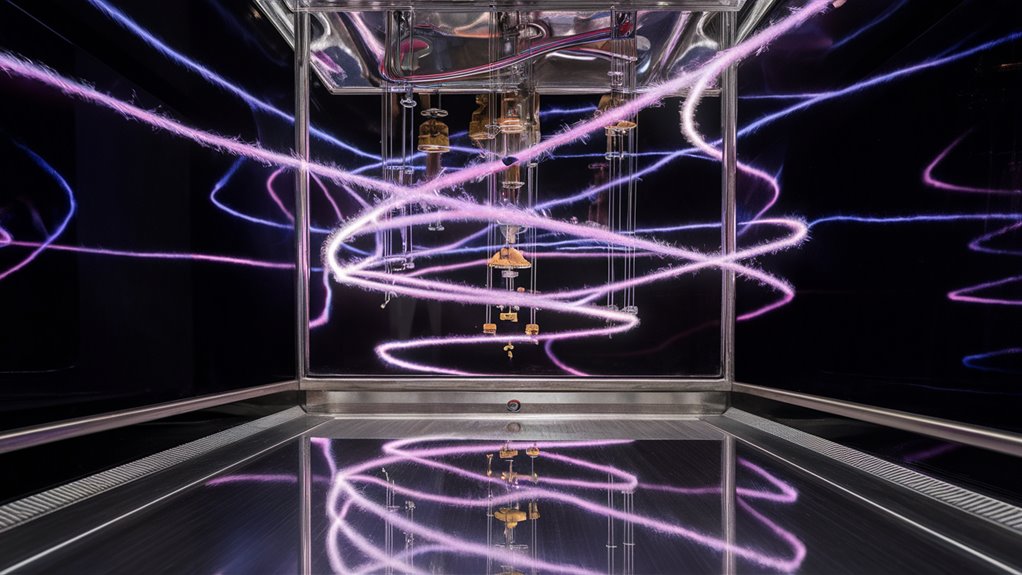
Signal Processing and Noise Reduction
It is the sophisticated trend in detection methods which makes possible the critical separation between true ionic binding events and background interference.
Coincidence detection: just those events which trigger multiple detectors inside given time windows
Clever signal processing algorithms artfully sift out spurious electronic signals from authentic temporal ionic interaction data, keeping the original intact.
Quantum Dynamic Analysis
Three parameters determine the analysis of ionic interaction: binding time, charge transfer rate, and energy exchange.
Advanced correlation function mapping reveals in-depth quantum dynamics. By integrating measured data with theoretical modeling, precise predictions on the ionic behavior in various electromagnetic media can be made, achieving new levels of accuracy in ionic interaction research.
Key Measurement Components
Time-resolved detection systems
High bandwidth oscilloscopes
Photomultiplier tubes
Coincidence detection hardware Foliage & Fortune
Signal processing algorithms
Correlation function analysis
Applications in Materials Engineering
Ionic Measurements in Materials Engineering: Microsecond applications
Breakthrough Technologies in Ionic Measurement
Microsecond ionic measurement technology has transformed today’s materials engineering.
By tracking precisely microsecond-level ionic interactions, engineers can now build materials with hitherto unprecedented accuracy.
These advanced measurement systems allow for real-time manipulation of molecular binding forces, creating adaptive surfaces with improved environmental sensitivity.
Advances in Smart Coatings
In materials science, smart protective coatings are also a significant advance.
Now protective layers are given ionic charge distribution control through the application of ion-lash principles.
These revolutionary materials now offer the following:
Active corrosion resistance
Automatic conductivity adjustment
Self-healing characteristics in the case of minor structural damages
Polymer Composite Advances
Control of the behavior of ion-lash has altered composite polymer engineering.
With microsecond precision measurement, the result is:
Fine-tuned molecular structure formation
Enhanced mechanical properties
More efficient manufacturing methods 먹튀검증업체
Saving on materials
Durable products of a higher quality
These technological breakthroughs have made possible materials that were impossible to try before, and marked a new era in material engineering capability.
The Future of Ion-Lash Technologies
Quantum-Controlled New Frontiers of Invention
Research into ion-lash technologies has revealed several key opportunities for development.
Development of Quantum Controlled Ion Gates are about to revolutionize atomic level charged particle manipulation.
Responsible control of ion-lash binding sites over time will allow unparalleled precision in the manipulation of molecular structures.
Priority Research Areas
Development of Hybrid Systems
Hybrid ion-lash systems integrating conventional binding theories with the entanglement properties of quantum physics offer a critical road forward. These achievements promise greater control and functionality across many different applications.
Strategies for Energy Efficiency
Exchange of Light Energy
Intermediate Device Efficiency
Indirect Typing
All layers offer much potential in reducing energy consumption, and in its overall level of availability. Our goal is to thus increase the efficiency as far as possible and improve sustainability.
Meeting of Machine Learning Algorithms
Real-time optimization coming from machine learning algorithms will bring a revolutionary change to ion-lash behavior prediction and control, enabling adaptive responses and improving performance metrics.
The Five Year Development Horizon
Development of nanoscale ion-lash devices will present new possibilities in fields such as targeted drug delivery and quantum computing. Key points of development are:
Enhancement of stability control
Advanced materials engineering – future development goal
Resistance to ion bombardment
The maintenance of structural integrity under extreme conditions
Optimization of Miniaturization in Practical Applications
Protocols for incorporating existing technologies
These advances will provide the basic infrastructure of posts for ionic brush applications in the next generation, overlapping many scientific domains at once.